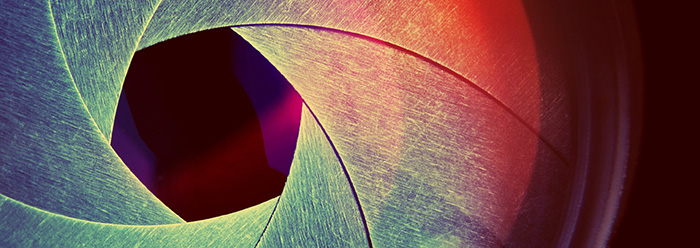
The term ‘tissue clearing’ describes a collection of techniques used for making large, fixed biological specimens transparent. Tissue clearing enables volumetric (three-dimensional) imaging of sample types such as thick tissue sections, organoids, and intact organs using light microscopy, and can even be applied for studying whole organisms.
Tissue clearing principles
Biological samples have a high water content and contain multiple structures formed of proteins and/or lipids. Each of these components has a different refractive index (the speed at which light travels through that component compared to the speed at which it travels through a vacuum), and it is the resultant heterogeneity within a sample which leads to a lack of transparency. Tissue clearing techniques aim to remove, replace, or alter some of the sample components to equilibrate the refractive index, thus rendering the biological specimen optically transparent.
Applications
Tissue clearing has become routine when imaging samples ranging from 100 μm to several centimeters in thickness. Compared to the established practice of slicing biological material into thin (~10 μm) sections for imaging, before piecing together the information obtained from adjacent slices, tissue clearing offers faster time to results and minimizes the risk of error.
Additionally, tissue clearing is seeing increased use to enhance the capabilities of super-resolution microscopy. For example, by developing an optimized clearing agent for high-resolution fluorescence imaging (SeeDB2), researchers have been able to image tissue samples at a depth of >100 μm1. This is reportedly an order of magnitude greater than was previously possible under standard mounting conditions.
Importantly, introducing a tissue clearing step into standard immunostaining protocols represents a means of enabling high-resolution imaging to a depth of several hundred microns with regular confocal microscopes2. A growing number of tissue clearing techniques are available to researchers, each with different advantages and limitations.
Tissue clearing techniques
Tissue clearing techniques can broadly be categorized according to the type of chemistry they use. Each of the available approaches has evolved over time to address common challenges.
Organic solvents serve to dehydrate samples and remove lipids, resulting in a refractive index of around 1.55, which is thought to be comparable to that of proteinaceous structures2. The main advantage of using organic solvents is that they provide rapid clearing, making their use compatible with immunostaining protocols. However, dehydration prevents most fluorescent proteins from maintaining emissions.
Methods designed to circumvent this problem include 3D imaging of solvent-cleared organs (3DISCO), in which the methanol dehydration step is replaced with incubation in tetrahydrofuran, and immunolabeling-enabled three-dimensional imaging of solvent-cleared organs (iDISCO), where methanol dehydration is followed by immunofluorescence with an antibody directed against the fluorescent protein in question3,4. These techniques have been shown to maintain fluorescent emissions for several days.
Aqueous-based tissue clearing involves submerging samples in a solution containing a high refractive index (1.44-1.52) molecule such as sucrose, fructose, or glycerol and leaving them to gradually become transparent5. Advantages of this approach include its simple protocol and the fact that (unlike using organic solvents) it enables direct lipid staining. However, aqueous-based tissue clearing can be prohibitively slow for larger samples, while the high viscosity of many concentrated sugar or glycerol solutions makes them difficult to work with.
Within the past decade, a number of lower viscosity aqueous clearing solutions have been developed. These include FocusClear™ and Histodenz™, both of which come with a fairly high price tag, as well as lower cost options such as 2,2′-thiodiethanol (TDE) and the FRUIT protocol. The latter is based on SeeDB2 (see above) and uses a cocktail of fructose and urea to synergistically clear samples including whole adult rabbit brains6.
Hyperhydration uses detergents to remove lipids from samples in conjunction with urea-mediated hydration, which lowers the refractive index to the 1.38–1.48 range2. By avoiding the use of organic solvents, hyperhydration maintain an aqueous environment for fluorescent proteins. However, its utility is limited by long clearing times, which make it impractical for integration into immunostaining protocols or processing large samples.
The first hyperhydration technique to be described (Scale) has since been superseded by ScaleS and other Scale family members7,8. These newer incarnations improve on the original method by being faster, avoiding tissue expansion, and preserving lipids. Another hyperhydration method, known as clear unobstructed brain imaging cocktails and computational analysis (CUBIC), includes an optional sucrose-based step to expedite clearing9. However, because CUBIC uses a high detergent concentration to maximize lipid removal, it suffers from significant protein loss5.
Hydrogel embedding stabilizes sample proteins by cross-linking them to a hydrogel. Any lipids are then removed using a detergent (or electrophoresis-based methods for faster lipid extraction) and the sample is submerged in a solution with a refractive index of 1.38-1.45. Although hydrogel embedding demonstrates excellent tissue clearing, detergent-based protocols are slow and electrophoresis-based methods can be difficult to implement2.
CLARITY is the earliest example of hydrogel embedding and remains in use today10. Within the past decade the original method has been modified to yield methods termed passive CLARITY technique (PACT), perfusion-assisted agent release in situ (PARS), active CLARITY technique-pressure related efficient and stable transfer of macromolecules into organs (ACT-PRESTO), and system-wide control of interaction time and kinetics of chemicals (SWITCH), all of which employ different strategies to shorten experimental workflows2.
Factors to consider
Whenever there is a need for imaging fixed samples more than a few tens of microns thick, it is prudent to consider tissue clearing. Choosing between the available methods should involve careful evaluation of sample size and whether lipid staining is required, as well as include determining whether the clearing method should be compatible with immunostaining. For further guidance, speak with antibody and clearing agent manufacturers, who can often share relevant product reviews or publications.
Jackson ImmunoResearch specializes in producing secondary antibodies for life science applications. Our portfolio encompasses a wide selection of products that are validated for common research applications, including immunofluorescence.
References
- Ke MT, Nakai Y, Fujimoto S, et al. Super-Resolution Mapping of Neuronal Circuitry With an Index-Optimized Clearing Agent. Cell Rep. 2016;14(11):2718-2732. doi:10.1016/j.celrep.2016.02.057
- Ariel P. A beginner’s guide to tissue clearing. Int J Biochem Cell Biol. 2017;84:35-39. doi:10.1016/j.biocel.2016.12.009
- Ertürk A, Becker K, Jährling N, et al. Three-dimensional imaging of solvent-cleared organs using 3DISCO. Nat Protoc. 2012;7(11):1983-1995. doi:10.1038/nprot.2012.119
- Renier N, Wu Z, Simon DJ, Yang J, Ariel P, Tessier-Lavigne M. iDISCO: a simple, rapid method to immunolabel large tissue samples for volume imaging. Cell. 2014;159(4):896-910. doi:10.1016/j.cell.2014.10.010
- Richardson DS, Lichtman JW. Clarifying Tissue Clearing. Cell. 2015;162(2):246-257. doi:10.1016/j.cell.2015.06.067
- Hou B, Zhang D, Zhao S, et al. Scalable and DiI-compatible optical clearance of the mammalian brain. Front Neuroanat. 2015;9:19. Published 2015 Feb 24. doi:10.3389/fnana.2015.00019
- Hama H, Kurokawa H, Kawano H, et al. Scale: a chemical approach for fluorescence imaging and reconstruction of transparent mouse brain. Nat Neurosci. 2011;14(11):1481-1488. Published 2011 Aug 30. doi:10.1038/nn.2928
- Hama H, Hioki H, Namiki K, et al. ScaleS: an optical clearing palette for biological imaging. Nat Neurosci. 2015;18(10):1518-1529. doi:10.1038/nn.4107
- Susaki EA, Tainaka K, Perrin D, et al. Whole-brain imaging with single-cell resolution using chemical cocktails and computational analysis. Cell. 2014;157(3):726-739. doi:10.1016/j.cell.2014.03.042
- Chung K, Wallace J, Kim SY, et al. Structural and molecular interrogation of intact biological systems. Nature. 2013;497(7449):332-337. doi:10.1038/nature12107
Learn more: | Do more: |
---|---|
Indirect and direct Western blotting | Exhibition Schedule |
Chemiluminescence western blotting | Cite and Win |
An Introduction to Expansion Microscopy | Past Competition Winners |