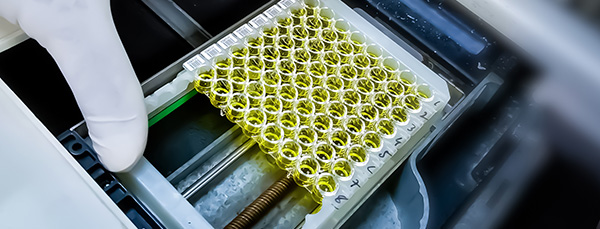
Antibodies are complex proteins produced by mammalian B cells during the adaptive immune response. Their unique ability to bind a broad array of targets with high specificity and affinity has been widely exploited for scientific research, and their use continues to grow. Here, we cover some antibody basics to help guide product selection.
Innate and adaptive immunity
Mammals have evolved two main lines of defense against potentially harmful pathogens or toxins. The first of these, innate immunity, includes anatomical barriers such as the skin and mucous membranes, secretions such as saliva and tears, and various mechanisms for identifying ‘non-self’ molecules (e.g., the complement system). The second, adaptive immunity, involves developing an immunological memory of a foreign invader, allowing for a more efficient response upon re-exposure. B cells and T cells are the major cell types involved in adaptive immunity. Of these, it is the B cells that are responsible for antibody production.
Antibody production by B cells
B cells are produced in the bone marrow before migrating to the spleen and entering the circulatory system. Each B cell expresses a different antibody (the B cell receptor) at its surface as a result of genetic mechanisms including V(D)J recombination, junctional diversification, and somatic hypermutation. When a B cell encounters its specific antigen, it differentiates to form short-lived plasma cells, long-lived plasma cells, and memory B cells. The short-lived plasma cells produce a secreted form of the B cell receptor to promote antigen clearance by the immune system before undergoing apoptosis (usually within 3-5 days). The long-lived plasma cells and memory B cells respectively reside in the bone marrow and circulation for many years and can mount a rapid immune response upon re-encountering the antigen.
Antibody structure
An antibody molecule consists of two identical heavy chains and two identical light chains, held together by disulfide bonds. The two antigen binding regions, known as the Fab fragments for ‘fragment antigen binding,’ both recognize the same epitope. They can be separated from the antibody molecule using papain, which generates two separate Fab fragments, or with pepsin, which removes the two conjoined Fabs in the form of a F(ab’)2 fragment. The effector end of the antibody, known as the Fc fragment for ‘fragment crystallizable,’ is highly conserved across antibodies of the same isotype. During an adaptive immune response, the Fc fragment interacts with Fc receptors (FcRs) on the surface of cell types, including macrophages, neutrophils, and dendritic cells, or with complement proteins, to drive processes such as antibody-dependent cell-mediated cytotoxicity (ADCC) and complement-dependent cytotoxicity (CDC).
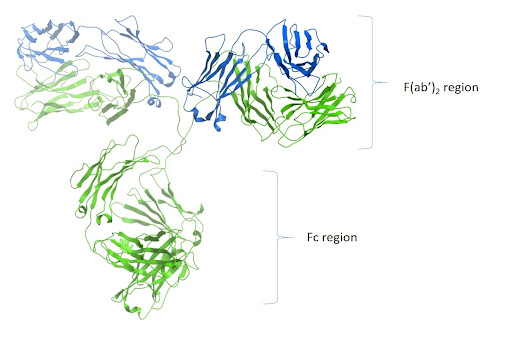
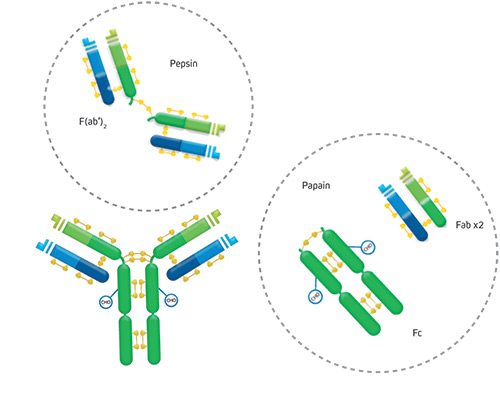
Antibody isotypes, sub-types, and valencies
Mammalian antibodies are classified into five main isotypes (IgA, IgD, IgE, IgG, and IgM) based on the type of heavy chain they contain (respectively alpha, delta, epsilon, gamma, and mu). Antibody heavy chains differ in terms of the number and amino acid sequence of the constant domains and the structure of the hinge region. For example, gamma heavy chains have three constant regions and a flexible hinge, while mu heavy chains have an additional constant region in place of a hinge region. Some antibody isotypes are further classified into sub-types by their heavy chain sequence. For example, human IgG has four sub-types (IgG1, IgG2, IgG3, and IgG4), which are numbered according to their relative serum abundance. In all mammals the light chains are classified as either kappa or lambda. Valency, a term describing the number of antigen-binding sites on a single antibody molecule, is another important variable. While IgG, IgD, and IgE are bivalent, IgA has a valency of 4, and IgM has a valency of 10.
Polyclonal and monoclonal antibodies
The terms polyclonal and monoclonal are widely used within the antibody manufacturing industry to describe the types of products being offered. To put this terminology into context, recall that each circulating B cell expresses a different antibody at the cell surface. When an animal is immunized with a protein, different B cells recognize different epitopes, giving rise to multiple B cell populations. If the blood is collected and the antibodies purified from the serum, the resultant product will contain antibodies from all of those B cell clones, hence the term polyclonal. In contrast, if the B cells are isolated from the blood and used to generate hybridoma via limiting dilution methods, each antibody preparation will be the product of a single clone and will therefore be monoclonal. A main advantage of polyclonal antibodies is that they can provide signal amplification, while monoclonal antibodies benefit from guaranteed long-term supply.
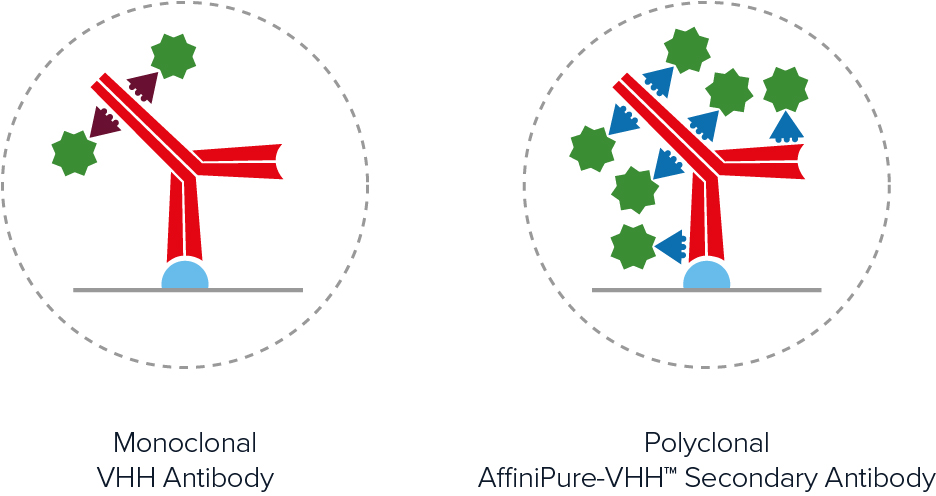
Recombinant antibodies
Recombinant antibodies differ from traditional polyclonal and monoclonal antibodies in that they are produced using recombinant methods. These involve obtaining the antibody sequence (e.g., by hybridoma sequencing, B cell sequencing, or using an antibody phage display library), cloning it into an expression vector, and using this to transfect mammalian cells that are grown in culture. The secreted antibody is then extracted from the culture medium. The advantages of recombinant antibodies include their high batch-to-batch consistency, guaranteed long-term supply (due to the antibody sequence being known), and amenability to engineering. On the flip side, because recombinant antibodies are monoclonal, they lack the capacity for signal amplification. To address this limitation, recombinant oligoclonal antibodies (mixtures of recombinant monoclonals) are being developed.
Primary and secondary antibodies
The main difference between primary and secondary antibodies is the types of biomolecules that they recognize and bind. While primary antibodies recognize protein antigens (e.g., cytoskeletal proteins, cell surface markers or signaling molecules), secondary antibodies recognize antibodies from a particular host species and of a specific isotype/sub-type. Secondary antibodies are generally produced in the same way as polyclonal primary antibodies, but using an antibody as the immunogen. For example, goat anti-rabbit IgG secondary antibodies are produced by immunizing a goat with rabbit IgG antibodies. Historically, primary antibodies were unlabeled and secondary antibodies bearing reporter molecules such as enzymes or fluorescent proteins were used for their detection. However, labeled primary antibodies are now widely available.
Direct vs. indirect detection
The use of secondary antibodies for detecting primary antibodies is known as indirect detection. A main advantage of this approach is that it provides signal amplification due to multiple secondaries binding each primary antibody (most secondary antibodies are polyclonal). This can be especially useful when monoclonal primary antibodies are being used to detect low-abundance antigens. The use of secondary antibodies also offers access to a broad range of reporter molecules, allowing researchers to be more flexible when planning their experiments, and can represent a more budget-friendly option than using labeled primaries. Advantages of direct detection are that it shortens experimental workflows by eliminating the secondary antibody incubation step and any associated washes, as well as can reduce the risk of non-specific signal resulting from secondary antibody cross-reactivities.
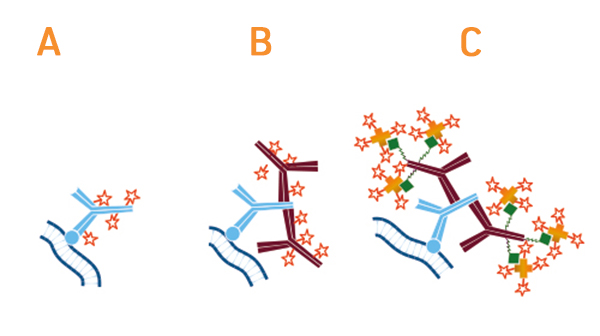
FabuLight™ secondary antibodies are labeled Fab fragments with specificity for the Fc region of IgG or IgM antibodies. They can be used for attaching fluorophores or biotin to primary antibodies prior to incubation with the sample, thereby eliminating the need for a secondary antibody incubation step yet still providing signal amplification.
Factors to consider for secondary antibody selection
The vast array of secondary antibodies that is available, coupled with their capacity for signal amplification, makes them a popular choice for many different research applications. However, there are several important factors that should be considered when selecting secondary antibodies, including the following:
Type of label
Secondary antibodies are labeled with enzymes such as horseradish peroxidase (HRP) and alkaline phosphatase (AP), fluorescent proteins such as R-phycoerythrin (RPE) and allophycocyanin (APC), and a broad range of fluorescent dyes (e.g., Brilliant Violet®, DyLight™, and Alexa Fluor® dyes). They may also be conjugated to biotin, gold nanoparticles or agarose beads, as well as many other moieties. Choosing between the available labels largely comes down to the type of immunoassay being performed and whether there is a need for multiplexing. For example, an ELISA for a single analyte might use a secondary antibody labeled with HRP to produce a chromogenic readout, and that same secondary antibody could also be used for chemiluminescent Western blotting, while a microscopy-based assay for detecting both a total protein and a phosphorylated isoform might use a pair of fluorophore-labeled secondary antibodies for multiplexed detection.
Cross-adsorption
Because antibodies from different host species often share similar structure and homology, a secondary antibody raised against the immunoglobulins of one species may also recognize those of another. This can result in non-specific signal due to off-target binding. One way of avoiding non-specific signal is to use cross-adsorbed secondary antibodies, which are secondaries that have been pre-incubated with species other than the target. Situations where cross-adsorbed secondary antibodies are especially useful include immunohistochemistry (IHC), to prevent unwanted secondary antibodies binding to the sample material; sandwich ELISA with indirect detection, to prevent secondary antibodies from binding to the capture antibody; and various multiplexed experiments, to safeguard accurate target detection.
Whole antibody or antibody fragment
Although intact secondary antibodies remain highly popular for scientific research, antibody fragments such as Fab and F(ab’)2 are seeing increased use. One reason for this is that they eliminate the risk of non-specific binding to Fc receptors on immune cells due to not having an Fc region. In addition, the smaller size of antibody fragments allows them to enter cells and tissues more easily than whole antibody molecules, making them useful for detecting intracellular targets.
Tertiary reagents
An alternative approach to using secondary antibodies for indirect detection is to introduce a tertiary reagent into the experimental workflow. For example, a biotinylated secondary antibody bound to an unlabeled primary antibody might be detected with a labeled streptavidin reagent. This offers another means of signal amplification, as well as provides greater experimental flexibility if the desired secondary antibody is not available commercially.
Antibody applications
Antibodies have utility for an almost limitless number of research applications. These include established methods like Western blotting, ELISA, and flow cytometry, as well as newer techniques such as super-resolution microscopy (SRM), surface plasmon resonance (SPR), and expansion microscopy. Antibodies are also used for diagnostic and clinical purposes, spanning everything from pregnancy testing, confirming viral infections, and testing for banned drug substances, through to treating conditions including cancer, hematological disease, and immune-related disorders.
Learn more: | Do more: |
---|---|
Indirect and direct Western blotting | Exhibition Schedule |
Chemiluminescence western blotting | Cite and Win |
An Introduction to Expansion Microscopy | Past Competition Winners |